Unveiling the Earth’s Fury: Understanding the Causes and Dynamics of Earthquakes
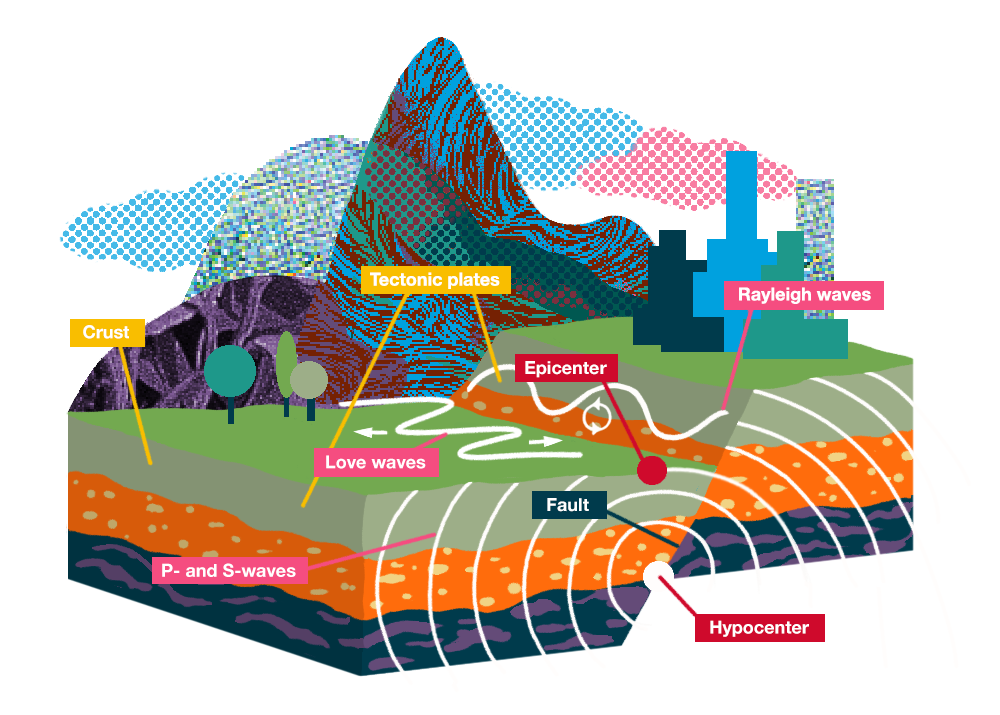
Introduction:
Earthquakes, with their awe-inspiring and often devastating power, are natural phenomena that have captivated human curiosity for centuries. These seismic events, born from the dynamic forces beneath the Earth’s surface, shape landscapes, alter coastlines, and, at times, bring about catastrophic consequences. In this comprehensive exploration, we delve into the fundamental causes of earthquakes, the intricate processes that lead to their occurrence, and the dynamic events that unfold during a seismic upheaval.
Section 1: The Earth’s Dynamic Interior
1.1 Earth’s Layered Structure:
To comprehend earthquakes, it is crucial to delve into the Earth’s layered composition. The planet is divided into distinct layers, including the solid inner core, liquid outer core, mantle, and crust. Each layer plays a role in the geological processes that give rise to seismic activity.
1.2 Tectonic Plates and Plate Boundaries:
The Earth’s rigid outer layer, known as the lithosphere, is fragmented into enormous pieces called tectonic plates. These plates float on the semi-fluid asthenosphere beneath them. The interactions between these plates, occurring at plate boundaries, are the primary drivers of earthquakes.
Section 2: Tectonic Forces and Stress Accumulation
2.1 Plate Tectonics:
The theory of plate tectonics, a cornerstone in understanding earthquakes, postulates that the Earth’s lithosphere is divided into major and minor plates that constantly move. These movements, driven by forces like mantle convection and gravitational pull, lead to interactions at plate boundaries.
2.2 Types of Plate Boundaries:
There are three primary types of plate boundaries – divergent, convergent, and transform. At divergent boundaries, plates move apart; at convergent boundaries, they collide; and at transform boundaries, they slide past each other. The interactions at these boundaries create stress within the Earth’s crust.
Section 3: Faults and Strain Accumulation
3.1 Faults and Fault Lines:
Faults are fractures in the Earth’s crust along which movement has occurred. The accumulation of stress along faults can result in sudden releases of energy, leading to seismic activity. Fault lines, which often mark the surface expression of faults, become focal points for earthquakes.
3.2 Elastic Rebound Theory:
The elastic rebound theory explains how stress builds up along a fault line, causing rocks to deform elastically until the stress exceeds the rocks’ strength. When this threshold is breached, the rocks undergo rapid elastic rebound, releasing stored energy in the form of seismic waves.
Section 4: Seismic Waves and Earthquake Generation
4.1 P Waves and S Waves:
Earthquakes produce seismic waves, and there are two primary types – P waves (primary or compressional waves) and S waves (secondary or shear waves). P waves travel faster and can pass through both solids and liquids, while S waves travel more slowly and only propagate through solids.
4.2 Focus and Epicenter:
The point within the Earth where an earthquake originates is called the focus or hypocenter. Directly above the focus on the Earth’s surface is the epicenter. The depth and location of the focus influence the characteristics of the seismic waves and the impact on the surface.
Section 5: Magnitude and Intensity
5.1 Magnitude:
Earthquake magnitude quantifies the energy released during an earthquake. The Richter scale, though historically significant, has been largely replaced by the moment magnitude scale (Mw) as it provides a more accurate representation of an earthquake’s size. Each unit increase on the scale corresponds to a tenfold increase in amplitude.
5.2 Intensity:
Intensity measures the effects of an earthquake at specific locations. The Modified Mercalli Intensity (MMI) scale ranges from I (not felt) to XII (total destruction). Unlike magnitude, intensity is subjective and varies depending on factors like distance from the epicenter, local geology, and building structures.
Section 6: Earthquake Effects and Damage
6.1 Surface Rupture:
In some earthquakes, particularly those along fault lines, the ground may rupture at the Earth’s surface. This surface rupture is a visible manifestation of the fault movement, leaving scars on the landscape.
6.2 Ground Shaking:
The primary effect of an earthquake is ground shaking, which can vary in intensity depending on factors like the earthquake’s magnitude, depth, and distance from the epicenter. Seismic waves radiate outward, causing the ground to vibrate and buildings to sway.
6.3 Secondary Effects:
Earthquakes often trigger secondary effects such as landslides, liquefaction (the temporary transformation of soil into a fluid-like state), tsunamis (large ocean waves caused by undersea earthquakes), and ground ruptures, amplifying the overall impact.
Section 7: Earthquake Preparedness and Mitigation
7.1 Early Warning Systems:
Advancements in seismology have led to the development of early warning systems that can provide seconds to minutes of advance notice before strong shaking reaches a location. These systems are crucial for minimizing casualties and damage.
7.2 Building Codes and Infrastructure Resilience:
Implementing and enforcing stringent building codes is vital in earthquake-prone regions. Earthquake-resistant construction techniques and retrofitting existing structures enhance their resilience to seismic forces, reducing the risk of collapse.
Section 8: Notable Earthquakes in History
8.1 The Great San Francisco Earthquake (1906):
The earthquake that struck San Francisco in 1906 is etched in history as one of the most destructive. The magnitude 7.8 quake, coupled with subsequent fires, resulted in widespread devastation, reshaping the city’s landscape and influencing seismic safety measures.
8.2 The Tohoku Earthquake and Tsunami (2011):
The Tohoku earthquake, with a magnitude of 9.0, triggered a massive tsunami that inundated the coast of Japan in 2011. The disaster highlighted the interconnected nature of seismic events and underscored the need for global cooperation in disaster preparedness.
Section 9: Ongoing Research and Future Perspectives
9.1 Seismological Research:
Ongoing research in seismology continues to enhance our understanding of earthquakes. Advances in technology, including the use of satellite imagery, GPS, and seafloor sensors, contribute to more accurate monitoring and assessment of seismic hazards.
9.2 Earthquake Prediction Challenges:
Despite significant progress, accurately predicting the exact time, location, and magnitude of an earthquake remains an elusive goal. The complex and dynamic nature of Earth’s processes poses formidable challenges to earthquake prediction.
Conclusion:
Earthquakes, with their ability to reshape landscapes and test the resilience of civilizations, emerge from the intricate dance of tectonic forces beneath the Earth’s surface. The causes of earthquakes, rooted in the movements of tectonic plates and the release of accumulated stress along faults, unfold a narrative of the Earth’s dynamic and ever-changing nature. As we strive to comprehend the complexities of seismic events, the knowledge gained from studying earthquakes becomes a cornerstone for developing strategies to mitigate their impact and build a safer, more resilient future in regions prone to seismic activity.